Science Sleuths: the Science that Shapes Diagnostic Tests: PCR, qPCR – What’s the Difference?
As we have discussed in two previous issues (PCR – what’s behind this commonly used acronym? and Wanted Dead or Alive – Does PCR supersede traditional techniques?) PCR, or the polymerase chain reaction, is an incredibly sensitive technique to detect DNA and is used extensively in diagnostic testing.
Initially, the PCR reaction was run for 40 cycles and the resulting DNA segments amplified were examined by gel electrophoresis to determine if the DNA of interest was present or absent. The number of reaction cycles, 40 in most cases, means that the reagents become exhausted and additional amplification slows or stops, hence the term ‘end-point’ PCR. The DNA of interest, or target DNA, might be a gene identifying Streptococcus equi subsp. equi (the causative agent for ‘Strangles’) or a gene identifying Equine Herpes Virus 1 (EHV-1). Figure 1 demonstrates what end-point PCR results might look like were we to run four samples, three of which were positive and one negative.
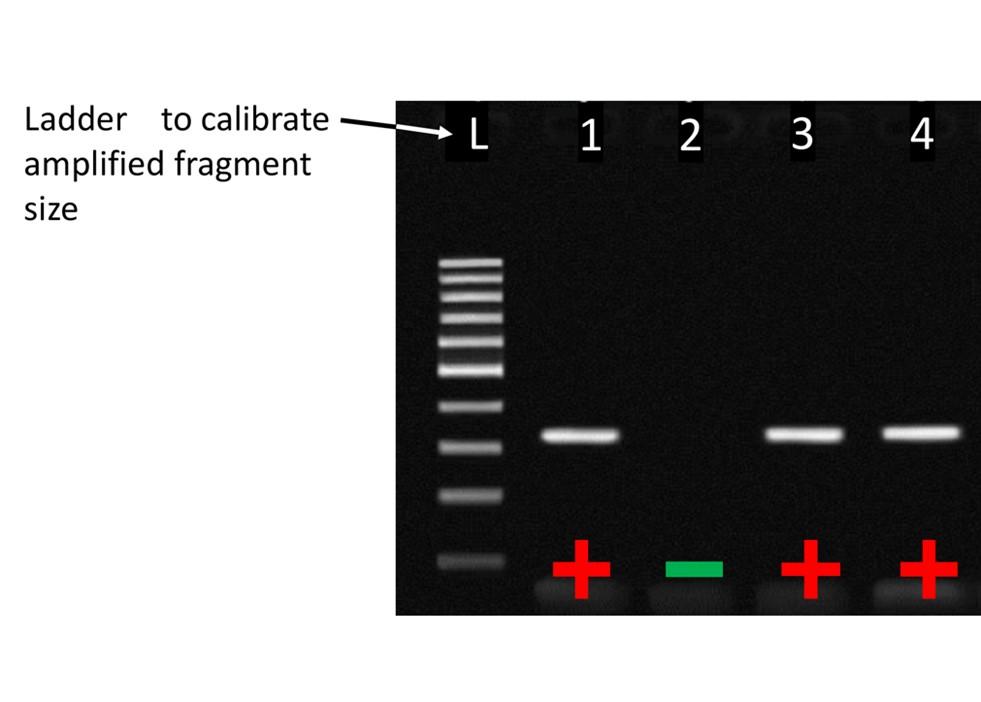
As technology has advanced, the ability to run PCR with automated protocols and read out semi-quantitative results has become available. These PCR methods have become known as Real-Time PCR or Quantitative PCR (qPCR). The many benefits of this more advanced technology include the ease with which it can be automated and scaled up, quick turnaround times and ability to roughly quantify the amount of target DNA of interest in the sample. For example, when testing for EHV-1, the question of approximately how much virus is present can be asked and help us understand if the quantity of virus particles being shed from its nasal passages or circulating in its blood stream.
A popular way to provide this semi-quantitative data is by having one of the reagents that make up the PCR reaction mix contain a fluorescent marker. This fluorescent marker is released when the reaction proceeds as target DNA is amplified. In this way fluorescence is detected at each amplification cycle of the reaction.
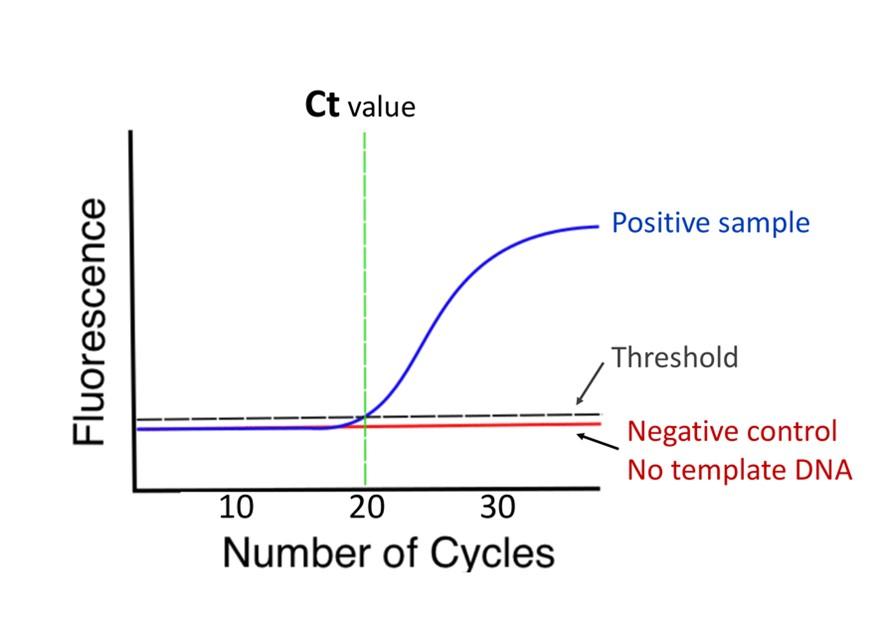
Logically, when a sample contains a large amount of target DNA, the reaction will become exponential in fewer numbers of reaction cycles than if the sample contained few target DNA templates. This concept is illustrated in Figure 3. To compare the relative quantity of starting material in samples a threshold is applied. This threshold is a level of fluorescence above which the sample is considered positive. The number of cycles of the reaction it takes to cross that threshold is the Ct value. For example, in Figure 2, the sample would be reported at Ct = 20, because it took 20 reaction cycles to cross the threshold. When we examine Figure 3, you can see that the larger the quantity of starting DNA template, the lower the Ct number.
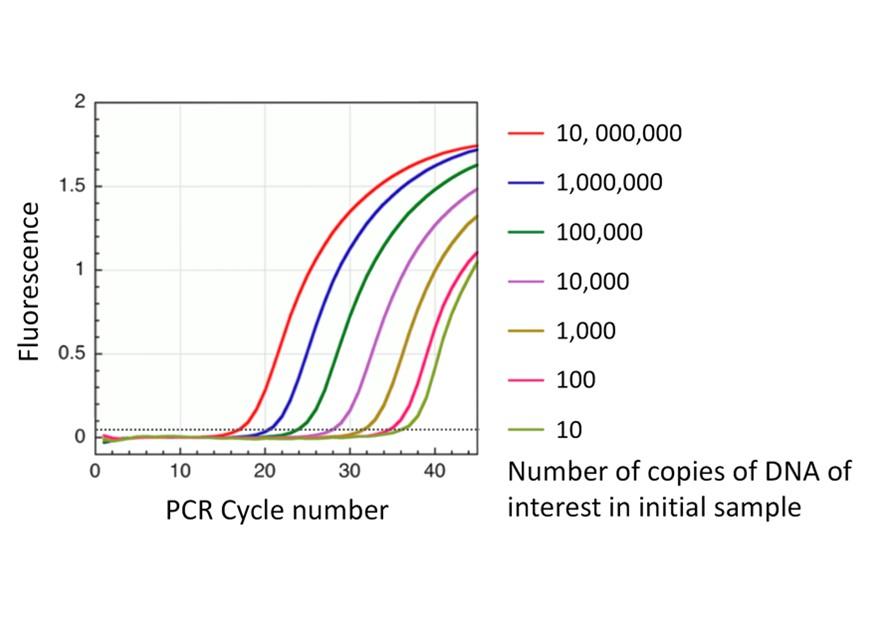
As PCR reactions proceed, mistakes are made – fidelity of DNA replication is never 100%. Statistically, the more cycles the reaction undergoes, the more likely errors are going to occur and off-target sequences amplified. With this in mind, much more research is needed to understand what positive results based on high Ct values (i.e., very low amounts of the target DNA) really mean in a biomedical context with regard to infection risk and disease control. However, whether we are thinking about animal or human infections, one thing is certain: proper biosecurity measures are essential for the control of any disease.
Emma Adam DVM, PhD, DACVIM, DACVS, is based at the University of Kentucky Gluck Equine Research Center and Veterinary Diagnostic Lab and is responsible for research and serve as a veterinary industry liaison. Jackie Smith, MSc, PhD, MACE, Dipl AVES, is an epidemiologist based at the University of Kentucky Veterinary Diagnostic Lab.